This article appears in the August 2022 issue of Front Vision, an educational Chinese-language magazine for kids. It is reproduced here with permission.
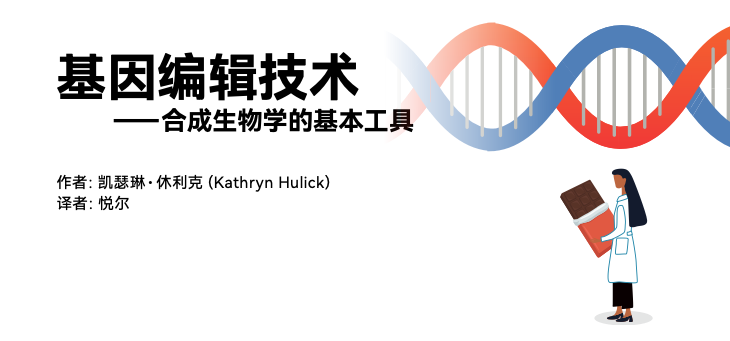
Inside a living cell, something remarkable is happening. A very tiny machine cuts the cell’s DNA. Next, the cell repairs the broken DNA, but the segment where the cut happened no longer works the same way. The tiny machine has edited the DNA—the code of life.
DNA is a long, spiraling molecule made of chemicals nicknamed A, T, C, and G. These chemicals are always paired: A with T and C with G. They are arranged into sequences called genes. Genes are sort of like the steps in a recipe to create a living thing and keep it healthy. Changing genes means changing the recipe. This will change how a living thing grows or develops.
CRISPR-Cas9 is a machine that can cut genes or parts of genes. “It is just like a pair of scissors,” says Joyce Van Eck, a plant biologist who works on biotechnology at the Boyce Thompson Institute in Ithaca, New York.
If you use scissors to cut the “add chocolate chips” step from a recipe for cookies and then then tape in “add raisins,” you end up with a raisin- filled dessert.
The scientists who developed CRISPR-Cas9, Jennifer Doudna and Emmanuelle Charpentier, won the 2020 Nobel prize in chemistry for their work. They first introduced this amazing machine to the world in June 2012. In her book about the groundbreaking discovery, A Crack in Creation, Doudna wrote, “We had built the means to rewrite the code of life.”
Scientists had found ways to change or edit genes before CRISPR-Cas9. In 2015, scientists cut and pasted a gene for “don’t grow horns” into cow DNA, and two new hornless cows named Buri and Spotigy were born.
The researchers who worked on these cows used an older method to edit the genes. These methods were all difficult, expensive, and time- consuming. CRISPR-Cas9 is easy, cheap, and quick. The invention of CRISPR- Cas9 ignited a revolution in biotechnology. The tiny machine has led to breakthroughs in medicine, agriculture, energy, conservation, and more.
What is CRISPR-Cas9? How does it work? Let’s find out.
Death to Viruses
Doudna and Charpentier didn’t build CRISPR-Cas9 from scratch. The inspiration for this machine came from nature. Bacteria need to defend themselves from invading viruses. So some bacteria use a system called CRISPR, which stands for Clustered Regularly Interspersed Short Palindromic Repeats.
That’s a mouthful of a name. It describes a segment of DNA that contains many short, repeating sections. These sections happen to be palindromes (that means they read the same forward and backward). They separate segments of DNA that the cell has taken from invading viruses and saved for later from each other. It’s like a catalog of enemies. If one of these viruses tries to invade again, the cell will recognize it as an enemy.
The bacteria’s CRISPR system also contains Cas genes, which hold the instructions for building Cas proteins. There are many different types of Cas proteins. All of them either unzip or cut DNA.
When an unknown virus attacks, the bacteria’s CRISPR defense system kicks into gear. It builds Cas proteins. These proteins look at the viral DNA for something called the PAM (protospacer adjacent motif). This mouthful of a name refers to a very short sequence of DNA that acts like a key or a guide. The Cas proteins cut out a section of viral DNA right next to that key. Then, they paste this stolen DNA into the CRISPR system.
When another virus of the same kind invades, the bacteria will copy the stored bit of viral DNA onto RNA, called CRISPR RNA, or crRNA for short. It also makes another type of RNA, called tracrRNA, which acts like a handle for the Cas protein to grab onto. The Cas proteins carry both types of RNA out to confront the intruder—the viral DNA.
Once again, the Cas proteins look for the PAM. When they find it, they check the viral DNA sequence right next to it. If the two sequences match, then the Cas proteins get to work breaking the DNA apart. This vanquishes the virus!
The CRISPR-Cas9 Machine
Doudna and Charpentier discovered that a particular type of Cas protein, Cas9, would cut DNA cleanly in the exact location matching the crRNA. They also found a way to join together crRNA and tracrRNA into one molecule, single- guide RNA, also called guide RNA or sgRNA. Perhaps the most important part of their work, though, was that they showed it was possible to customize this guide RNA to seek out any sequence of DNA, even the DNA inside a living human, animal, or plant cell. Scientists could choose what gene to cut and where to cut it. This is what made the discovery so incredible.
What happens after CRISPR-Cas9 cuts DNA? The cell repairs the damage on its own. But mistakes may happen, so a gene that has been cut and repaired will sometimes be disabled. This is called a gene knock-out. A gene that has been knocked out no longer works correctly. So it will no longer be able to control traits in the same way.
Sometimes, scientists want to add a new or modified DNA segment to replace the one that CRISPR-Cas9 destroyed. To do this, they design a new strand of DNA called donor DNA. It must have short sections at each end that match the ends on each side of the section of broken DNA. “It’s kind of like delivering cargo using two docking arms,” says Van Eck. After DNA has been cut, the cell is “looking for something to insert,” she says. It will find the donor DNA and paste it into the broken spot. This is called a gene knock-in.
Scientists may want to knock out or knock in multiple genes at once. This is called multiplexing. It’s easy with CRISPR-Cas9 because all scientists have to do is introduce separate guide RNA molecules (and separate donor DNA, if desired) for each gene they want to edit.
When they made the discovery, Doudna and her colleagues realized that scientists could use CRISPR-Cas9 to change the recipe for any living thing. This tiny machine could make it possible to fix genetic diseases. It could alter the traits of animals and plants or even people. It was the perfect tool for gene editing.
On the evening that Doudna learned that her team’s experiments with CRISPR-Cas9 had worked, she felt elated. “Visions of this tiny machine danced in my head. Suddenly, I found myself laughing out loud. How incredible that bacteria had found a way to program a warrior protein to seek and destroy viral DNA! And how miraculous that we could repurpose this fundamental property for an entirely different use. It was a precious time of pure joy, the joy of discovery.”
The Recipe for Life
Living things don’t come with instruction manuals. To change a trait or cure a disorder using gene editing, researchers first need to identify which genes control that trait or disorder. The first step to figuring this out is to sequence the genome. This means figuring out the entire DNA code of a living thing, letter by letter. This is a monumental task—the human genome contains three billion letters arranged into approximately twenty-four thousand genes. It took thirteen years for researchers to completely sequence the first human genome, a feat that concluded on April 14, 2003. Thanks to advances in technology, it now takes just one or two days to sequence a human genome. However, the genomes of most plant and animal species have not yet been sequenced.
In addition, pinpointing which genes control which traits isn’t easy. Often, many genes control one trait, and one gene may contribute to multiple traits. For example, some chickens have a mutated gene that frizzles their feathers—they curl outward instead of lying flat. This mutation has quite a few effects not at all related to feathers. Two examples are that chickens with the mutation eat more and lay fewer eggs than typical chickens.
All of this means that changing one gene often is not enough to change a trait. This is especially true for vague traits such as skill at sports or intelligence. Samuel Sternberg is a biochemist at Columbia University and worked with Doudna to develop CRISPR-Cas9. He says, “Most traits we think about are the result of tens or hundreds or thousands of variants, each one of which contributes a fraction of a percent to that trait.” He goes on to explain that your DNA alone does not determine exactly who you are or how you develop. Your environment and the relationships you have matter too. “The way genes are expressed can be impacted by the way an organism grows up,” he says.
In addition, changing one gene may have effects beyond what scientists expect or desire. Scientists must perform many experiments on cells in the lab to figure out the complex relationships between genes and traits. After a lot of work, researchers determine a problem gene and a way to change it for the better. For example, in 2016, researchers at Penn State University used CRISPR-Cas9 to snip a single gene out of the white button mushroom. Without this gene, the mushroom can’t turn brown as quickly when it gets cut. It stays fresh longer. CRISPR-Cas9 can easily knock out this gene in a mushroom cell in a dish in a laboratory. But a living mushroom contains millions of cells. How is it possible to make enough edits?
One way to do this is to edit germ cells, which include sperm and egg cells. A genetic edit made in a germ cell will spread to all of the body cells as the plant, animal, or fungus grows. Another way to do it is to use viruses to carry CRISPR-Cas9 material into stem cells (which can grow into any type of cell) or other body cells. In plants, scientists typically use bacteria to edit the DNA of a single leaf cell in the lab. Then they can coax that cell to grow into an entire plant.
CRISPR in the Real World
Doctors have already used gene therapy to cure sickle cell disease. In this disease, a genetic defect causes red blood cells to grow in a crescent shape instead of a circle. The irregular shape causes blood to clump and block blood vessels, which can be painful or even fatal. Now, doctors can take a sample of stem cells from the person’s blood and edit the genes to correct the defect. Then they deliver the corrected stem cells back into the body, where the cells start churning out new, healthy blood cells. The procedure is still new and going through testing, but it has already improved the lives of many patients.
Gene therapy could also cure heart disease and help cure or treat many other diseases and disorders ranging from cancer to COVID-19. Heart disease is the condition that causes the most deaths worldwide. In July 2022, a patient went through the world’s first experimental procedure of this type. Doctors injected a version of CRISPR into the patient’s body. This CRISPR machine had been designed to turn off a single gene named PCSK9. This gene controls a person’s level of LDL cholesterol, sometimes called bad cholesterol. When too much of this stuff builds up in the body, heart attacks and heart disease become more likely. Without the gene, LDL levels should stay low. “If this works and is safe, this is the answer to heart attack—this is the cure,” says Sekar Kathiresan, CEO of Verve, the company running the experiment.
In agriculture, CRISPR is leading to animals and plants that are healthier, tastier, or easier to grow or raise. Van Eck notes that CRISPR has greatly sped up the time it takes to develop improved crops. This is especially important as climate change is likely to lead to food shortages. Many genetic changes, such as the knocked-out mushroom browning gene, could have happened through natural mutations and selective breeding. But it takes a long time for a mutation to occur naturally.
Van Eck’s team is using CRISPR-Cas9 to edit tomato plants. One goal they have is to make plants more compact. Some tomato plants grow up to six feet (two meters) tall and branch out a lot. A smaller plant that produces just as many tomatoes would be “easier for farmers to grow,” says Van Eck. In addition, more compact tomato plants could be grown indoors in growth chambers. Indoor gardens aren’t as vulnerable to climate change.
Back in 1928, farmers discovered a natural mutation that resulted in a more compact tomato plant. In 2019, Van Eck’s team used CRISPR-Cas9 to knock out a single gene and cause that same mutation. They still need to do more research to make sure the plants are hardy and will do well in growth chambers. But they’ve already tasted them. “They taste the same,” says Van Eck. “We always taste what we work on.”
CRISPR is everywhere in science. It is being used to develop allergy-free foods, mosquitoes that can’t spread malaria, coral that can survive climate change, algae that do a better job producing biofuels, vaccines for diseases including COVID-19, and much more. It is even being used to try to resurrect traits of the extinct woolly mammoth.
Like all technology, CRISPR is neither good nor bad. It is a single tool in the vast toolbox that is genetic engineering. What matters is how people use this and other tools. In the right hands, CRISPR can help lead to scientific breakthroughs that will reduce suffering and improve our world.